The role of energy in regional disparities: Decentralized renewable energy and livelihood security of Amazonian Indigenous communities
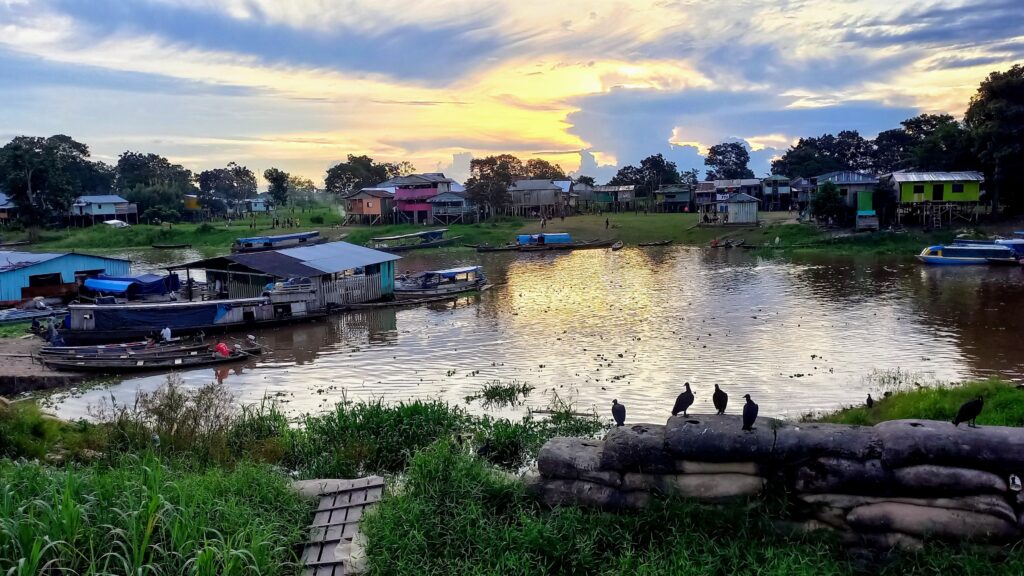
By Nora Hampl, Department of Political Science, University of Vienna, Universitätsstraße 7, 1010 Vienna, Austria (ORCiD; e-mail).
Cover Photo, Leticia on the Amazon River (Department of Amazonas, Colombia; border of Colombia, Brazil and Peru) is courtesy of Hampl, Romão.
Research context
Several Amazonian countries have updated their Nationally Determined Contributions (NDCs) to achieve carbon neutrality by 2050. Pledges were made to halt deforestation and achieve emission reductions in the highest emitting sectors. Land-based mitigation measures account for up to 70% of the NDC’s mitigation capacity in some countries. It is broadly acknowledged, however, that mitigation and adaptation strategies cannot come at the cost of biodiversity or cultural loss, even when confronted with complex cross-sectoral challenges (IPCC, 2022). Therefore, the no-harm imperatives inscribed in mitigation and adaptation strategies must translate into policies safeguarding against inappropriate human interventions (Kramarz et al., 2021), particularly in rural areas where such measures will have the highest impacts. Additionally, if decarbonization and mitigation in the energy and mining sectors remain as weak as they currently are, the negative externalities will once again be largely felt by rural communities, including remote Indigenous communities living in the Amazon bioregion.
Short-term rural adaptation livelihood approaches in the region have mostly not been translated into long-term sustainability and resilience, posing uncertainties for future generations of Amazonian Indigenous populations. Combined with other stressors (e.g., large-scale development, environmental crime and the expansion of illicit economies into both protected areas and Indigenous lands) (Abreu et al., 2019), the goal of preserving the Amazon biome as a “bioculture” – a “habitat” for non-human species as well as a “home” of culturally diverse Indigenous communities – is gradually disappearing from mankind’s imaginaries (Pereira & Viola, 2021). An average of 2.3 km2 of the Amazon is destroyed every hour, even though an estimated 90,970 tons of living plants are found on each km2. The encroachment on the Amazon forest and subsequent cycles of deforestation-drought-fire-further forest loss (Gatti et al., 2021; Lapola et al., 2023) have benefitted specific industries (i.e., sub-soil extractives, industrial agriculture, or the meat industry—particularly in Brazil’s states of Roraima and south-eastern Pará), raising questions as to who decides what constitutes “success” or “good outcomes” in the Amazon bioregion (i.e., out-migration, large-scale extractive development or intensive industrial agriculture?).
The Amazon bioregion
The Amazon biogeographic region expands beyond the larger Amazon River basin and comprises diverse ecosystems, including rainforests, seasonal forests, deciduous forests, flooded forests, savannas and others. Hundreds of distinct ethnic groups, including no-contact Indigenous tribes, reside across nine South American countries and nine Brazilian states (Figure 1). Given the biocultural and ecological diversity, no single renewable energy system design can match the different cultural and ecological contexts.
Figure 1. International and Brazilian Amazon (% of Amazon each country contains / % of natural forests)
Source: Author’s own elaboration, forest data from WRI, RAISG.
Because Brazil contains 61.8% of the Amazon, it has been the focal point of efforts to save the biome and its biocultures. However, the smallest countries that cumulatively contain only a negligible percentage of the Amazon (Guyana 2.5%, French Guiana 1%, and Suriname 1.7%), are also countries with the highest natural forest cover (89%, 97.3%, 94.2%, respectively), therefore likely highest levels of biodiversity and species density. Retaining the natural forest cover intact is critically important for the overall health of the biome and requires coordinated efforts at the Pan-Amazonian regional level.
Logics across scales
Renewable energy technology has been deployed at different scales and for different purposes—as an add-on to existing energy-intensive infrastructures or to achieve commercial and industrial objectives at scale (carbon credit and/or profit maximization). At the local scale, a renewable energy system can be designed to improve local livelihoods and address vital human needs. The purposes implicit within scales may be incompatible, for example, when large-scale commercial and industrial projects are deployed regardless of whether they generate burdens locally (i.e., negative externalities) or whether they breach local protocols and traditional land tenure regimes. Combined with an ad hoc application of FPIC (i.e., free, prior and informed consent) (Ewell, 2022) and lack of transparency in benefit-sharing, sustainability and energy transitions, particularly in peripheral regions of the Global South, are more often than not referred to as inequitable. However, thicker descriptive accounts of what equitable sustainability transitions and equitable futures might look like for Amazonian Indigenous communities tend to be left out of both practitioners’ and researchers’ imaginaries.
The definition of equitable proposed in this study is twofold – first, scaled-down renewable energy solutions need to focus on meeting the vital needs of rural communities over the long term so that more resilient and equitable futures can be envisioned and built upon. Second, in the Amazon, the terms of self-determined development (UNDRIP, Art. 23) need to be aligned with climate and ecological imperatives (i.e., saving the biome), which requires navigating through slippery trajectories of multi-scalar (and cross-sectoral) sustainability and energy strategies. To situate equitability and extrapolate the trade-offs between climate ambition, development priorities, and local livelihoods, a mixed qualitative-quantitative analysis of scales becomes useful in answering questions such as – did large-scale development projects (e.g., hydroelectric plants or grid extensions) improve local livelihoods, or rather, decreased water availability locally while providing electricity supply for new extraction sites, or cross-border electricity trade? Have large-scale renewable energy projects that source renewables locally, but are owned extra-locally, generated any benefits for local indigenous communities? Have they addressed local human security needs, and if so, how? On the one hand, renewable energy deployed at the utility scale is rapidly becoming the main route to decarbonize electricity matrices, increase energy supply for energy-intensive industries, or maximize cross-border electricity trade—all while helping to achieve NDCs. On the other hand, rural development practitioners concerned with poverty alleviation at the sub-national level have thus far not fully taken advantage of the multiple ways in which renewable energy can be deployed to address human security needs and improve prospects for self-determined development. Moreover, public funding continues to support fossil fuel policies except in Brazil (Figure 2).
Figure 2. Public funds committed to recovery packages via energy-sector policies (Colombia, Brazil and Peru). (January 2020 to January 2021, in $ millions)
Source: Author’s own elaboration, funding data sourced from: energypolicytracker.org; License: Creative Commons Attribution CC BY-NC-SA 4.0.
Synergies between renewables, decarbonisation, and land/forest strategies
Diversified portfolio of small-scale decentralized energy resources (DER) using renewable energy (RE) tailored to local contexts has several advantages (Javid et al., 2021). First, it can reduce over-reliance on hydropower. Second, in rural areas with limited or no access to electricity, a combination of renewable sources and storage technologies can provide a stable energy supply without increasing emissions while cutting industrial pollution (Alonso et al., 2020), and when done right, without eroding biodiversity. Third, current reliance on fossil-fuel energy for residential and productive purposes can be reduced if not completely eliminated. Furthermore, electrification on the end-use side (transport, cooking, cooling/heating) results in higher energy efficiency.
Decentralized renewable energy solutions with storage. Mini-grids and stand-alone systems using local renewable resources present a less costly alternative, while providing an opportunity to transition to more sustainable livelihoods (from pesticide and chemical-fertilizer-based farming to agro-ecology using organic fertilizer, from transported, costly fossil fuels to locally sourced renewables, with lower exposure to health hazards).
Renewables and energy efficiency. Clean power generation leads to efficiency improvements and should be coupled with reductions in primary energy demand, resulting in significant energy-related carbon-emission reductions (IRENA, 2017).
Renewable potential. Total primary energy supply (TPES) in the Amazon region remains dominated by non-renewables. New additions to renewable electricity capacity are still dominated by large-scale hydropower, while not enough solar is being added.
Complementarity. The significant share of hydropower in the existing regional electricity matrix provides opportunities to develop complementarities with other renewable energy technologies and, simultaneously, achieve both the cost-efficiency and reliability of power systems. Solar power is notably under-represented in the current electricity generation and capacity but rising sharply in Brazil (though not in the Amazon).
Solar, wind, biomass. Indicators of renewable resource potential are high for biomass, wind, and solar PV, and can be rapidly scaled across sectors, and integrated into local circular local bio-economies. Converting mini-grids to hybrid solar systems (using solar, hydro, and biogas technologies), and combining them with storage technologies (electrochemical batteries, thermal, mechanical, compressed air, hydraulic (pumped), or electric field storage (capacitors) make it possible to integrate different energy sources and reduce the risk of power outages.
Biomass potential (net primary production) is high. Anaerobic digestion of agriculture and livestock residual waste is estimated in some countries, potentially making up 25% of national electricity generation and 90% of the demand for natural gas and LPG (Gutiérrez et al., 2022). Biogas and bioLPG derived from bio-waste could replace up to 90% of the demand for petroleum-derived natural gas and LPG. In remote Amazonian villages, forest biomass was proposed as a viable, sustainable energy supply (Brandão et al., 2021). However, a closer look at ongoing research is warranted since methane emissions along biomethane and biogas supply chains were found to be grossly underestimated (Bakkaloglu et al., 2022).
Waste-to-energy. Capture and reuse strategies can significantly contribute to emission reduction mitigation in the waste sector (UN World Water Development Report, 2017). Capturing and using biogas for energy from waste streams and treating domestic wastewater contribute to emission reductions, particularly in more densely populated urban areas.
Deforestation, biodiversity loss and cultural loss. Efforts to halt deforestation and protect the megadiverse Amazon biome, should be a joint national and global ambition. Without disturbing biodiversity-rich ecological systems, locally-sourced clean renewables (sun, wind, river, biomass) could open up clean energy access for millions living in the Amazon still without energy or only with access to costly imported fossil fuels (mostly diesel). Livelihoods on Indigenous reserves, community collectives and designated farmlands would exponentially improve (Table 1). Because off-grid systems can be tailored to adapt to local needs and conditions, they can be a particularly good option for Indigenous reserves and protected areas, where the aim is to protect the intactness of both the cultural and ecological systems with a minimal carbon footprint.
Table 1. Benefits of on-sight renewable energy generation & phased-out fossil fuels
Source: Author’s elaboration based on document analysis (Marine pollution bulletin, Journal of Cleaner Production, Environmental Epidemiology, Energy Research & Social Science, Environmental and Experimental Botany
Conclusions
While the NDC ambitions of Amazonian countries are well articulated, they risk inappropriate human intervention unless coordinated across scales and sectors and focus on the well-being of populations living in rural areas and remote, otherwise neglected regions. Policy recommendations outlined in this study focus on energy solutions that prioritize clean power generation and energy efficiency improvements. These can, first and foremost, alleviate some of the harms felt by local communities (i.e., toxic landscapes, air pollution, lack of access to health care, and physical safety) (HRW, 2021). Additionally, they can boost prospects for self-determined livelihood trajectories based on climate-compatible land/forest use (circular bio-economies). In this way, distributed renewable energy solutions can contribute to long-term sustainability and resilience and emission-reduction mitigation stated in NDCs.
Recommendations
References
IPCC (Intergovernmental Panel on Climate Change). 2022. Climate Change 2022: Mitigation of Climate Change. (accessed 6 May 2022).
Kramarz et al., Governing the dark side of RE: A typology of global displacements. Energy Research & Social Science 2021 (74), 101902.
B.R. de Abreu, G.W. Siqueira, H. Liebl, M.H. Nascimento, Difficulties in the control of environmental crimes in the Amazon, Int. j. adv. eng. res. sci. 6 (5) (2019).
J.C. Pereira, E. Viola, Climate change and biodiversity governance in the Amazon: At the edge of ecological collapse?, Routledge, 2021.
L.V. Gatti, L.S. Basso, J.B. Miller, M. Gloor, L. Gatti Domingues, H.L. Cassol, G. Tejada, L.E. Aragão, C. Nobre, W. Peters, L. Marani, Amazonia as a carbon source linked to deforestation and climate change, Nature, 595 (7867) (2021) 388-393.
D.M. Lapola, P. Pinho, J. Barlow, L. E. Aragão, E. Berenguer, R. Carmenta, H.M. Liddy, H. Seixas, C.V. Silva, C.H. Silva-Junior, A.A. Alencar, 2023. The drivers and impacts of Amazon forest degradation. Science. 379 (6630), eabp8622.
Ewell, Reimagining the Renewable Energy Transition: The Potential for Mandatory Corporate Due Diligence to Ensure Respect for the Right to Free, Prior, and Informed Consent, Yale j. int. law47 (2022) 309.
UNDRIP United Nations Declaration on the Rights of Indigenous Peoples. 2007.
Javid et al., Futuristic decentralized clean energy networks in view of inclusive-economic growth and sustainable society. Journal of Cleaner Production 2021 (309), 127304.
D.L. Alonso et al., Assessment of mining activity on arsenic contamination in surface water and sediments in southwestern area of Santurbán paramo, Colombia. Journal of environmental management 2020 (264).
IRENA (International Renewable Energy Agency). 2017. Synergies between renewables and energy efficiency.
A.S. Gutiérrez et al., Potential of livestock manure and agricultural wastes to mitigate the use of firewood for cooking in rural areas: The case of the department of Cordoba (Colombia). Development Engineering 2022 (7).
P.C. Brandão, A.L. de Souza, P. Rousset, F.N.B Simas, B.A.F. de Mendonça, Forest biomass as a viable pathway for sustainable energy supply in isolated villages of Amazonia, Environ. Dev. 37 (2021) 100609.
Bakkaloglu, J. Cooper, A. Hawkes, Methane emissions along biomethane and biogas supply chains are underestimated, One Earth 5 (6) (2022) 724-736. DOI: 10.1016.
UN World Water Development Report. 2017. Wastewater: The Untapped Resource. (accessed 6 May 2022).
HRW (Human Rights Watch). Colombia. Events of 2021.